Description
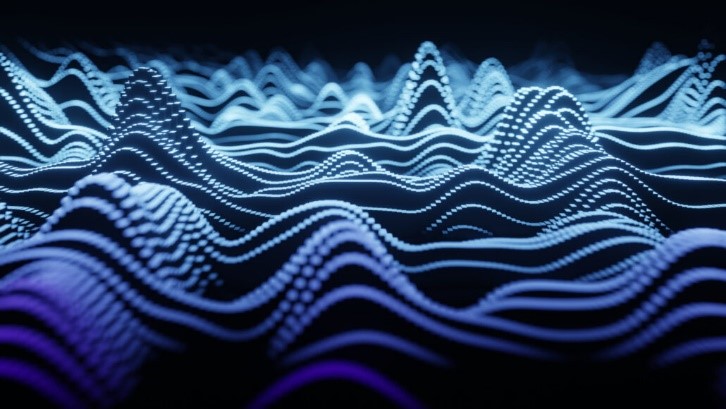
Disclaimer: Copyright infringement not intended.
Context
- In the new study, the researchers developed an acoustic beam-splitter – a tiny device resembling a comb – that could both emit and detect individual phonons.
Phonons: Background and Description
- For the engineers who design cell phones, solar panels and computer chips, it’s increasingly important to be able to control the way heat moves through the crystalline materials — such as silicon — that these devices are based on.
- In computer and cell-phone chips, for example, one of the key limitations to increasing speed and memory is the need to dissipate the heat generated by the chips.
- To understand how heat spreads through a material, consider that heat — as well as sound — is actually the motion or vibration of atoms and molecules: Low-frequency vibrations correspond to sound, while higher frequencies correspond to heat.
- At each frequency, quantum mechanics principles dictate that the vibrational energy must be a multiple of a basic amount of energy, called a quantum, that is proportional to the frequency. Physicists call these basic levels of energy as phonons. In a sense, then, “phonon” is just a fancy word for a particle of heat.
Phonons in Crystals
- Phonons are especially relevant in the behavior of heat and sound in crystals.
- In a crystal, the atoms are neatly arranged in a uniform, repeating structure; when heated, the atoms can oscillate at specific frequencies. The bonds between the individual atoms in a crystal behave essentially like springs.
- When one of the atoms gets pushed or pulled, it sets off a wave (or phonon) travelling through the crystal.
- In practice, most materials are filled with a chaotic mix of phonons that have different frequencies and are traveling in different directions, all superimposed on each other.
.jpeg)
Defining Phonons
Phonons are collective atomic vibrations, or quasiparticles, that act as the main heat carriers in a crystal lattice. The very quietest sounds of all consist of individual — and indivisible — phonons.
Types of Phonons
Acoustic Phonons and Optical phonons
- When the unit cell contains more than one atom, the crystal will contain two types of phonon, acoustic and optical.
- Optical phonons are excited easily by light. In acoustic phonons, both positive and negative ions swing together. In optical phonons, positive and negative phonons swing against each other.
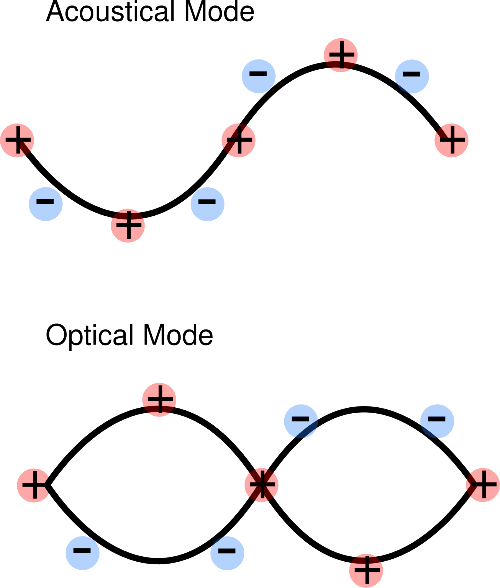
Phonons and Photons
- The way Photons are packets of light energy; similarly, phonons are packets of vibrational energy.
- Like photons, phonons are bosons and not conserved; they can be created or destroyed in collisions.
- Unlike photons (the particles that carry light or other electromagnetic radiation), which generally don’t interact at all if they have different wavelengths, phonons of different wavelengths can interact and mix when they bump into each other, producing a different wavelength. This makes their behavior much more chaotic and thus difficult to predict and control.
- Just as photons of a given frequency can only exist at certain specific energy levels — exact multiples of the basic quanta —so, too, can phonons.
- Unlike photons, which can travel through empty space, phonons need a medium such as air or water — or in the case of the new study, the surface of an elastic material.
Extra Information
- In the quest for better ways to dissipate heat from computer chips — a key requirement as chips get faster and pack in more components — finding ways to manipulate the behavior of the phonons in those chips, so the heat can be removed easily, is the key.
- Conversely, in designing thermoelectric devices to generate electricity from temperature differences, it’s important to develop materials that can conduct electricity (the motion of electrons) easily, but block the motion of phonons (that is, heat).
Recent Research: Building Quantum Computer with Phonons
- There is a question if can we build a quantum computer whose information unit is, sound.
- According to a paper published in Science recently, it should be possible.
- Phonons can’t be permanently broken into smaller bits. But, as the new experiment showed, they can be temporarily divided into parts using quantum mechanics.
- Earlier the problem was that researchers can manipulate electrons using electric currents, magnetic fields, etc., and they can manipulate photons with mirrors, lenses, etc. – but what can they manipulate phonons with? This always remained a puzzle.
- To this end, in the new study, researchers from the University of Chicago have reported developing an acoustic beam-splitter.
Beam-Splitter
Beam-splitters are used widely in optics research. Imagine a torchlight shining light along a straight line. This is basically a stream of photons. When a beam-splitter is placed in the light’s path, it will split the beam into two: i.e. it will reflect 50% of the photons to one side and let the other 50% pass straight through.
While it seems simple, the working of a beam-splitter actually draws on quantum physics. If you shine a million photons at it, it will create two beams, each of 500,000 photons. We can then reflect these two beams to intersect each other, creating an interference pattern (recall Young’s double-slit experiment). But researchers have found that an interference pattern appears even when they shine photons at the beam-splitter one by one. What are the photons interfering with? The answer is themselves.
This is because a) particles can also behave like waves, and b) until an observation is made, a quantum system exists in a superposition of all its possible states (like a qubit being partly ‘on’ and partly ‘off’ at the same time). So when the single wave interacts with the beam-splitter, it enters a superposition of the two possible outcomes – reflected and transmitted. When these states recombine, an interference pattern shows up.
|
Acoustic Beam-Splitter
- An acoustic beam-splitter is a tiny device resembling a comb, with 16 metal bars jutting out of it. It was placed in the middle of a 2-mm-long channel of lithium niobate.
- Each end of the channel had a superconducting qubit – a qubit whose circuit components were superconducting – that could both emit and detect individual phonons. The whole setup was maintained at an ultra-low temperature.
- If these phonons were converted to sound, their frequency would be too high for humans to hear. Each phonon in the study represented, the “collective” vibration of around one quadrillion atoms.
- The team found that these phonons interacted with the comb just like photons interact with an optical beam-splitter.
- When a phonon was emitted from the left side of the channel, it was reflected half of the time and transmitted to the right side the other half. When phonons were emitted simultaneously from the left and the right sides, they both ended up on one side (as expected).
In a nutshell,
- Acoustic beam splitter is a device that allows about half of an impinging torrent of phonons to pass through while the rest get reflected back. But when just one phonon at a time meets the beam splitter, that phonon enters a special quantum state where it goes both ways at once. The simultaneously reflected and transmitted phonon interacts with itself, in a process known as interference, to change where it ultimately ends up.
- The lab demonstration of the effect relied on sound millions of times higher in pitch than humans can hear, in a device cooled to temperatures very near absolute zero. Instead of speakers and microphones to create and hear the sound, the team used qubits, which store quantum bits of information.
- The researchers launched a phonon from one qubit toward another qubit. Along the way, the phonon encountered a beam splitter.
- Adjusting the parameters of the setup modified the way that the reflected and transmitted portions of the phonon interacted with each other. That allowed the researchers to quantum mechanically change the odds of the whole phonon turning up back at the qubit that launched the phonon or at the qubit on the other side of the beam splitter.
- A second experiment confirmed the quantum mechanical behavior of the phonons by sending phonons from two qubits to a beam splitter between them. On their own, each phonon could end up back at the qubit it came from or at the one on the opposite side of the beam splitter.
- If the phonons were timed to arrive at the beam splitter at the exact same time, though, they travel together to their ultimate destination. That is, they still unpredictably go to one qubit or the other, but they always end up at the same qubit when the two phonons hit the beam splitter simultaneously.
- If phonons followed the classical, nonquantum rules for sound, then there would be no correlation in where the two phonons go after hitting the beam splitter. The effect could serve as the basis for fundamental building blocks in quantum computers known as gates.
Thus, Phonons could lead to new quantum applications. But it’s still a long way from here to a functional quantum computer that uses phonons as units of information.
WHAT ARE QUBITS?
Quantum computers use qubits as their basic units of information. A qubit can be a particle – like an electron; a collection of particles; or a quantum system engineered to behave like a particle. Particles can do funky things that large objects – like the semiconductors of classical computers – can’t because they are guided by the rules of quantum physics. These rules allow each qubit to have the values ‘on’ and ‘off’ at the same time, for example.
The premise of quantum computing is that information can be ‘encoded’ in some property of the particle, like an electron’s spin, and then processed using these peculiar abilities. As a result, quantum computers are expected to perform complicated calculations that are out of reach of the best supercomputers today.
Other forms of quantum computing use other units of information. For example, linear optical quantum computing (LOQC) uses photons, the particles of light, as qubits. Just like different pieces of information can be combined and processed by encoding them on electrons and then having the electrons interact in different ways, LOQC offers to use optical equipment – like mirrors, lenses, splitters, waveplates, etc. – with photons to process information.
In fact, any particle that can be controlled and manipulated using quantum-mechanical phenomena should, on paper, be usable as an information unit in a quantum computer.
|
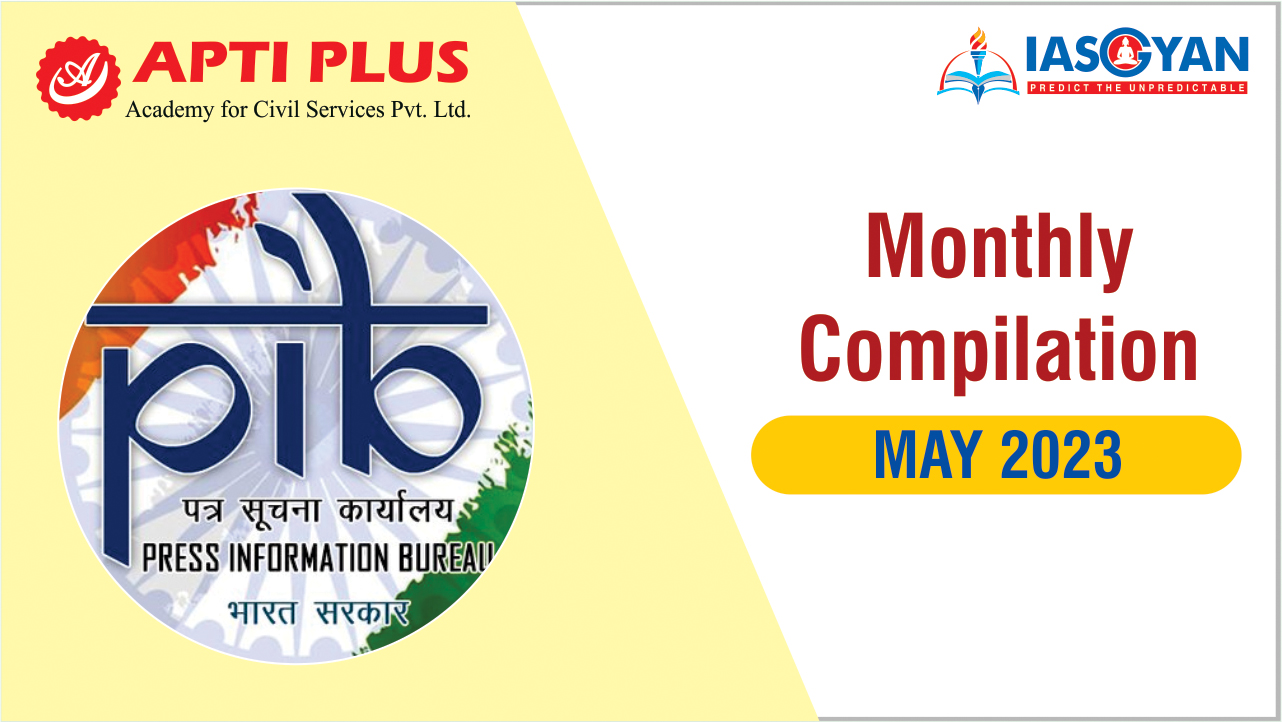
PRACTICE QUESTION
Q. Consider the following statements with respect to Phonons:
1. Phonons can travel through empty space.
2. Phonons are bosons and not conserved; they can be created or destroyed in collisions.
3. In acoustic phonons, both positive and negative ions swing against each other.
4. Phonons of different wavelengths can interact and mix when they bump into each other, producing a different wavelength.
Which of the above statements is/are correct?
(a) Only 1 and 3
(b) Only 2 and 4
(c) 1, 2 and 4
(d) All of the above.
Correct Answer: (b) Only 2 and 4
|
https://www.sciencenews.org/article/quantum-mechanics-sound-split-phonon